The recommendations for health maintenance, disease prevention, diagnosis, and treatment that we receive throughout our lives are largely based on our health characteristics. In addition, healthcare providers have always strived to make their practices more effective. For example, by experimenting with different treatments, observing, and sharing results and improving the efforts of previous generations. In the past, the possibilities were limited, but now technologies such as precision medicine are emerging.
Catching up with Moore
Advances in computing power and genetics and the growing availability of (health) data offer an opportunity to make accurate personalised patient care a clinical reality (Hodson, 2016). The first human genome was sequenced in 2001. After more than a decade and at a cost of about US$3 billion, Genome sequencing has become much faster and cheaper (Wetterstrand, 2020). This cost reduction led to accelerated innovation in genomics, enabling research on a larger scale.
Many genomes can now be sequenced in a single day for about $1,000 each. Many claims have been made, including by the National Human Genome Research Institute (NHGRI), that genomics outperforms developments in computing as measured by Moore’s Law (see Figure below).
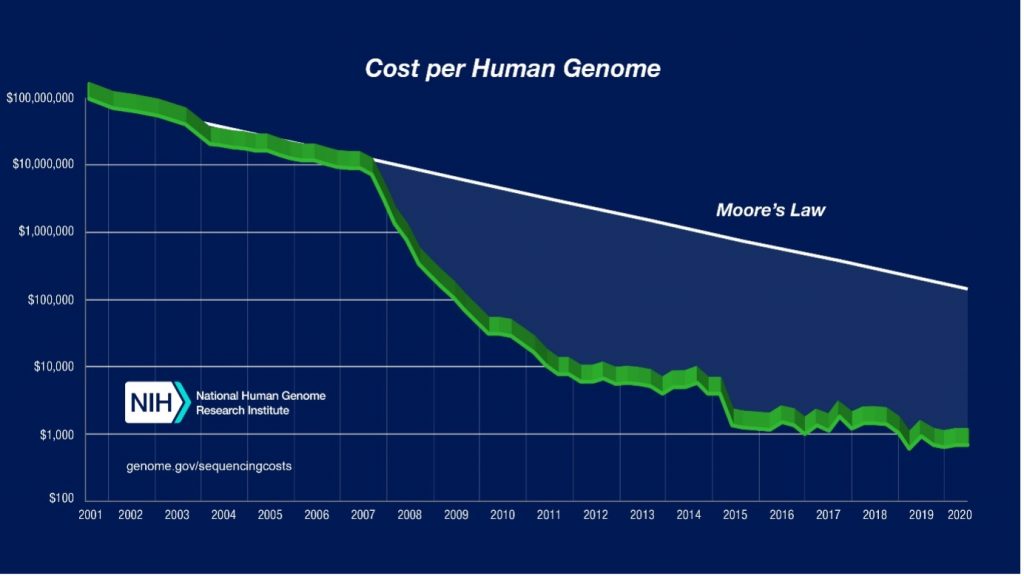
This law states that the speed of computers, measured by the number of transistors that can be placed on a single chip, will double every year or two and is considered the engine of the electronic revolution (Mollick, 2006). This means that computers are increasingly providing computing power to analyse the vast amounts of data generated by DNA sequence analysis or electronic health records (EHR).
Development and possibilities
This development has led genome sequencing to enter medical practice, particularly to diagnose rare conditions where conventional techniques have failed. For example, cancer, neurodegenerative diseases and rare genetic disorders take a huge toll on individuals, families and societies as a whole. Many of these conditions can be prevented through lifestyle changes, such as improving weight and diet, or reducing alcohol consumption. However, many are also caused by ethnic, racial, or familial factors (Bresnick, 2018). Discovering how a person’s genetics affect his or her chance of developing or surviving a particular condition would fundamentally revolutionise the way health care providers approach the practice of medicine.
As former President of the United States Barack Obama said in 2015: “I want the country that eliminated polio and mapped the human genome to lead a new era of medicine, one that delivers the right treatment at the right time”. In that same year President Obama launched the Precision Medicine Initiative (PMI). Meanwhile, the global healthcare industry is slowly shifting towards a proactive and predictive approach, using algorithms for predicting risk with a higher degree of precision in certain cancers and heart diseases from available clinical data (Ehteshami Bejnordi, 2017). Although current approaches to precision medicine in oncology have been fruitful, they require better integration and utilisation of available resources to inform sustainable and effective drug development and clinical care (Marabella, 2021). These resources consist of large quantities of genetic, clinical, social, lifestyle and preference data across broad, heterogenous populations. In turn algorithms can mine knowledge from the accumulated data. To this vast store of personal data, add clinical data captured by EHRs and DNA data captured through whole-genome sequencing, and precision care becomes realisable (Baker, 2019).
More customised healthcare instead of a one-size-fits-all approach, which is the case these days, could reduce the burden of disease by personalisation, and thus the cost of preventable health care for all. In the case of the latter, the healthcare industry must make it accessible to everyone. It is also considered that certain populations will not benefit from this innovative technique because some treatments will not work for those populations, or because they will be unaffordable. Like a tailored suit, it is not for everyone but the ones who fit will benefit.
References
Hodson, R., 2016. Precision medicine. Nature Portfolio. Available at: https://www.nature.com/articles/537S49a
[Accessed 29 September 2021].
Wetterstrand, K.A., 2020. The Cost of Sequencing a Human Genome. National Human Genome Research Institute (NIH). Available at: https://www.genome.gov/about-genomics/fact-sheets/Sequencing-Human-Genome-cost
[Accessed 29 September 2021].
Mollick, E.R., 2006. Establishing Moore’s Law. Institute of Electrical and Electronics Engineers: Annals of the History of Computing. Available at: https://www.researchgate.net/journal/IEEE-Annals-of-the-History-of-Computing-1934-1547
[Accessed 30 September 2021].
Bresnick, J., 2018. What Are Precision Medicine and Personalized Medicine?. HealthITAnalytics. Available at: https://healthitanalytics.com/features/what-are-precision-medicine-and-personalized-medicine
[Accessed 30 September 2021].
Ehteshami Bejnordi, B. et al., 2017. Diagnostic Assessment of Deep Learning Algorithms for Detection of Lymph Node Metastases in Women With Breast Cancer. National Library of Medicine: National Center for Biotechnology Information. Available at: https://pubmed.ncbi.nlm.nih.gov/29234806/
[Accessed 1 October 2021].
Marabella, C., 2021. Novel Immunotherapy Options Prompt Paradigm Shift in NSCLC Treatment. OncLive. Available at: https://www.onclive.com/view/novel-immunotherapy-options-prompt-paradigm-shift-in-nsclc-treatment
[Accessed 1 October 2021].
Baker, D.B., 2019. Precision Medicine: The Promise and the Challenges. The Healthcare Information and Management Systems Society (HIMSS). Available at: https://www.himss.org/resources/precision-medicine-promise-and-challenges
[Accessed 1 October 2021].
Figure ‘Cost per Human Genome’ credits: https://www.genome.gov/about-genomics/fact-sheets/Sequencing-Human-Genome-cost
Featured image credits: Vincent Yau